Leader
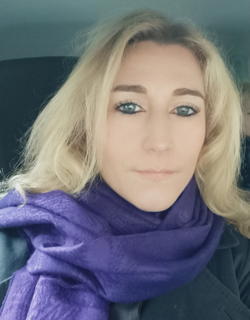
Dr.
Amélie KOCHEM CNRS Researcher
Chemistry and Biology of Metals laboratory
CEA-Grenoble
17 avenue des Martyrs
38 054 Grenoble Cedex
Tel. : 04 38 78 24 87
Non permanent staff of the team
Gaëtan Quintil, PhD Student, 10/2022 – 09/2025
Léa Diebold, Master 2 Student, 01/2023 – 07/2023
Gaëtan Quintil, Master 2 Student, 01/2022 – 07/2022
Ikram Yagoub, PhD Student, 10/2018 - 02/2022
Klara Schotte, Master 2 student, 01/2021 – 07/2021
Alicia Beaufils, Master 2 student, 01/2020 – 07/2020
Klara Schotte, Master 1 student, 04/2020 – 07/2020
Guillaume Delattre, Master 2 student, 01/2019 – 07/2019
Romain Bucci, Internship ENS Lyon, 06-07/2018
Research project
Context and positioning
Catalysis is cornerstone in chemistry. Many industrial chemical processes are indeed catalyzed. In this context, transition metal catalyzed processes have enabled many developments in organic synthesis. However, the power and efficiency of such methods are limited by the conventional focus on chemical reactions as discrete events. Therefore, the quest for new methodologies allowing the
straightforward,
cost-effective and
sustainable synthesis of
valuable and complex molecular targets such as pharmaceuticals
ingredients from simple precursors continues to be a great challenge for the scientific community.
In that domain,
Nature is a perfect model and an inexhaustible source of inspiration. Millions of years of evolution have produced biological systems capable of efficient one-pot multi-step catalysis, by using abundant and non-toxic metals, that give complex molecules in high yields. “One-pot” catalytic processes allow greater
economy of
time,
energy maximize resources and a
decrease in
material loss arising from multiple iterations of sequential reactions, workup and purification. Performing one-pot reactions also permits
thermodynamic levelling by coupling multiple catalytic cycles. Moreover, the grafting of homogeneous catalysts on surface to facilitate their reuse and recycling is also important to increase the atom efficiency of the whole process.
Synthetic chemists are beginning to draw inspiration from Nature for designing
one-pot (single reactor) processes involving multiple transformations followed by a
single workup stage. However, homogeneous catalysis is still
dominated by the use of
metals such as palladium, platinum, rhodium or ruthenium that are performing two electron transfer reactions. It is worth noting that developing multicatalysis with
rare and
potentially toxic metals does not meet the criteria of sustainable synthetic process. In that domain, iron might be considered as a “champion” due to its
abundance,
low cost, and relatively
non-toxic character. Considering that pharmaceuticals are the target molecules of this proposal, iron has another remarkable advantage. Drug substances can contain up to 1,300 ppm residual iron due to its low toxicity whereas it has to be ≤10 ppm for most other transition metals.
The usual formal oxidation states of iron range from –II to +IV allowing it to perform reaction involving multi-electron transfer. However, single electron transfer is always competitive and, in many cases, preferred. Once again,
Nature reveals itself as a true master regarding the
powerful strategies that it developed in controlling the type of redox action (1e− versus 2e−) by the mean of well-chosen ligands and metal/ligand or metal/metal cooperativity. Such cooperativity is based on the use of a redox partner (redox active ligand or redox active metal) involved in the catalytic cycle. In other terms, developing bio-inspired catalysts capable of such cooperativity is a powerful strategy to give a noble-metal character to iron.
We focus on the development of sustainable multicatalytic reactions by using catalysts based on iron and non-innocent ligands. Our goal is to deliver sustainable reaction pathway to access pharmaceutical active compounds.
The mean research axes developed in the group are described in the following figure.
Figure. Research axes investigated for the sustainable synthesis of drugs.

Axis 1 and 2:
Catalytic mechanism investigation: “better understanding for rational improvement” of known and new cascade reactions
The goal is this research axis is to offer a better understanding of the one-pot synthetic processes described in the literature to allow their rational improvement. The inability to rationally tailor the catalysts properties derives in part from a lack of fundamental knowledge. To address these issues we work on the establishment of the catalytic mechanisms involved as well as on the structure-dependent catalytic properties understanding. For this purpose, we take advantage of the power of combined spectroscopic, electrochemical and computational chemistry studies. These investigations offer a unique way to rationally tune the molecular catalyst (ligand design) and improve their efficiency.
In particular, we are interested in iron(cyclopentadienone) catalysts. These complexes have found numerous applications in the last years notably by providing unique reactivity for dihydrogen transfer and borrowing hydrogen. Borrowing Hydrogen enables the direct functionalization of saturated substrates such as alcohols, without the requirement for activation by stoichiometric oxidation to the corresponding reactive unsaturated compounds. It involves a metal-catalysed initial dehydrogenation (a), addition of the nucleophilic reagent NuH (b), and a final back hydrogen transfer (c), avoiding the waste associated with the distinct three-steps sequences classically required for such transformations. This is exemplified below with N-alkylation of alcohol.
Figure. Sustainable one-pot multicatalysis for the synthesis of pharmaceutical active compounds
Major achievements were recently reported regarding the efficiency and the regio- and chemo-selectivity of these catalysts based on
abundant and
non-toxic metal, but
further improvements are still
necessary. For instance, the use of additive (stoichiometric equivalent of strong base for instance) and elevated temperature are still necessary. The development of catalysts operating in milder (room temperature to improve the enantioselective transformation efficiency) and additive-free conditions (to increase the atom economy) is still desired. Moreover, the homogeneous condition renders the reuse and recycling of the catalyst difficult. The international scientific groups working in this field mainly focus on the development of new catalysts and the evaluation of their catalytic performances whereas fundamental questions about the mechanism remain. The questions that come at this stage are: how can be rationally improved the catalyst efficiency and the reaction conditions? How can be modified the catalyst to heterogenize it while keeping a high efficiency? The study of the catalytic mechanism offers a unique way to answer these questions. Thanks to these studies we develop more efficient catalysts based rational ligand design for known reactions and apply them for the discovery of new reactivity in collaboration with Dr. Adrien Quintard (SERCO team/DCM/Grenoble-Alpes-University).

Axe 3:
Molecular Fe-catalysts grafted on graphene-based materials (G-mats) as support and/or co-catalysts “reusable catalyst/new cascade reactions”
Over the time, numerous methodologies were developed to graft molecular catalysts on different supporting materials such as amorphous silica, alumina or zirconia oxides, as well as porous materials with high surface area providing a high catalyst loading and a possibility to use confinement effects (zeolite, mesoporous silicas, and layered structures). In order to get a higher gain, our goal is to graft molecular catalysts on a surface that can act as a supporting material (reusable heterogeneous catalyst) but also a catalytic partner (“non-innocent” material). Graphene materials can act as “non-innocent” support and are identified as an ideal candidate for this task.
Graphene (G) is a bidimensional layer of one-atom thick sp2 carbons in a hexagonal arrangement (fig.1-c). As such, it is the thinnest, the strongest, and the best semi-conducting material among all known materials.
Graphene material (G-mat) include G but also
graphene oxide (GO) obtained by deep oxidation of graphite and subsequent exfoliation of the resulting graphite oxide,
reduced GO (rGO) obtained from the generally not complete reduction of GO and
doped graphene material (doped-G-mat) in which hetero-elements are introduced. Since the discovery of single-layer G in 2004, research in the domain of G-mat met an explosive growth.
We focus on the grafting of iron molecular catalysts used in axis 1 and 2 on different type of G-mats as illustrated in the following figure. It benefits from the collaboration established with the
SyMMES/CAMPE team (CEA-Grenoble) specialized in the synthesis and characterization of G-mats. Our goal is to deliver reusable catalysts to push forward the sustainability of the reaction pathway we are interested in to access pharmaceutical active compounds.
Figure. Different type of graphene-based materials depending on their composition (G-mats are represented as single monolayer for the sake of simplicity)
Figure. Pictorial representation of supramolecular grafting of molecular catalysts (MC) on G-mats.